By James Mutegi, Intissare Mouamine, Hakim Boulal, Kokou Adambounou Amouzou, Jhon B. Valencia, Thomas Oberthür, and Simon Cook
Vision
Food systems around the world must sustainably increase their performance to meet the growing demand for the foods and ecosystem services they provide. This issue is global in nature but is particularly sensitive in Africa because food systems there, which have hardly improved over the previous 50 years, are being looked upon to meet surging regional, continental, and global needs. Our vision for agriculture is to move ever closer to satisfying a booming world demand for food while sustaining the system on which food production depends. Smallholder farms contribute to 80% of the overall food production in Africa, and around 300 million people who are dependent on these small-scale farms frequently encounter food insecurity (ILRI, 2022). The population in Africa has experienced substantial growth and projections estimate it will reach 2.5 billion by 2050 and around 4 billion by 2100 (ILRI, 2022; UN population, 2022). The significant impact of climate change on agricultural productivity and food security in developing countries in Africa is also widely recognized (Mutengwa et al., 2023).
Therefore, our goal is to develop a productive and sustainable agriculture that enables investment into these systems. This is accomplished by optimizing the interactions between climate, soil, plants, and management at the agricultural landscape scale according to the resources available to smallholder farms. Mitigating the impacts of climate change faced by these food-insecure smallholders involves both innovative and traditional indigenous land farming techniques including integrated soil fertility management practices, conservation agriculture, land restoration, and effective water management strategies (Mutengwa et al., 2023).
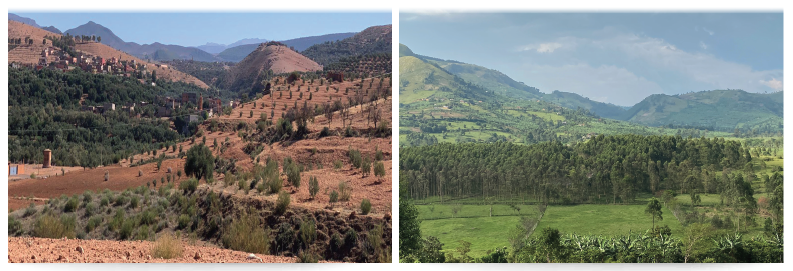
We aim for an agricultural system that captures solar energy and allows biological and chemical elements to sustain food production with efficient use of nitrogen (N) and phosphorus (P) that does not overload the environment, and with a reversal of the historic losses of soil carbon (C). Sustainable crop management practices optimize climate-soil-plant interactions, enhance nutrient use efficiency (NUE), and contribute to C sequestration into the soil ecosystem (Meena et al., 2020). Such an approach is conducive to stimulate and reward on-farm innovation to meet society’s demands for food, and its goals for water and C management.
As custodian of the 4R Nutrient Stewardship approach, the African Plant Nutrition Institute (APNI) is well positioned to operationalize such use of plant nutrition within innovative agronomy. According to Zingore and Johnston (2013), the 4R model has allowed farmers in various regions of the world to sustainably improve their yields, incomes, and livelihoods. Nitrogen and other fertilizer inputs (including manure) are managed to increase uptake and substantially support increases in biomass, water, land, and labour productivity through an expanded 4R concept. We envision this to improve green water (i.e., rainwater and soil moisture) productivity through a combination of water management and crop and pasture productivity gains. The enhancement of the agricultural sector holds the potential to elevate incomes and opportunities, empowering individuals to better cope with unforeseen challenges (Kray et al., 2022). In turn, coupled improvement in nutrient use efficiency and water productivity provides a platform for increased C sequestration within systems. We term this approach the nitrogen water carbon nexus (NWC). Furthermore, we envision sustainability in the agricultural landscapes to transcend field and farm boundaries into living agricultural landscapes.
Proposition
Water, carbon, and nitrogen must be managed jointly in landscapes, to maximize positive interactions, minimize trade-offs, and improve socio-economic well-being and resilience (Mutuku et al., 2017). Water is the controlling variable for N and C flows and processes, so knowledge on the flow of water through landscapes is key to understanding the substantial variation that exists (Erkossa, et al., 2015). Nutrition is the most readily managed and is key to stimulating biological production. About 65% of the degradation on African land since 1965 has been due to soil and nutrient exhaustion (WRI, 2001). A lot is known about dual interactions between one and another of these three components (NWC), but little is known about the mechanisms that drive interactions between all three of them at the landscape scale, and how they vary in the landscapes.
First, consider how water can be used better over agricultural landscapes. Water productivity (i.e., the conversion of water to benefit) must improve to avoid potential conflicts between users, and between the needs of the environment. In some places, it is largely a consequence of sustainable intensification (SI), which, as implemented, has not been a balanced fit given the limits of the surrounding ecosystem services (Tilman et al., 2011). Improved water productivity can mitigate the vulnerability of impoverished communities to natural disasters, including droughts (Cai et al., 2013). Adopting a sustainable water use strategy in Africa is essential to cope with climatic risks, economic shocks, and rising food prices (WRI, 2001). In Africa, the vulnerability of food systems to climate change is pronounced, given the predominant reliance on rainfed production and a generally low adaptive capacity across the continent (The African Climate Foundation, 2023). Water productivity of rainfed systems rarely exceeds 10-20% of its potential due to water scarcity, land degradation, and erosion of many landscapes (Koutroulis et al., 2019). Water pollution from agricultural runoff and throughflow is widespread because of inappropriate use of agrochemicals and livestock overloading.
Second, think about C. Water and C dynamics in landscapes are closely coupled (Shrestha et al., 2012). Retaining water in landscapes increases the probability of building up soil organic C through improved biomass production. Reducing overland flow reduces the risks of losing soil C.
Finally, take account of the N that is about to be added to landscapes. Nitrogen fertilizer use will increase over Africa in coming years, as will livestock densities. With a global warming potential of almost 300 (viz 1 kg N2O∝ 300 kg CO2 eq), the consequences of failing to manage this input are substantial. On the other hand, if N inputs are managed well and used productively the benefits are substantial, too. As indicated by Schut and Giller (2020), optimal agronomic efficiency leads to a favourable return on investment from fertilizer use among African smallholders. Despite the adoption of new technologies and interventions, a notable proportion of Africans continue to grapple with food security challenges (Ozor et al., 2013).
Now consider our proposal to bring together these components into a single coherent conceptual framework to explain the interactions of the three components: Carbon [C], Nitrogen [N], and Water [W]—if reordered from most manageable [N] to the least [C]—the NWC Framework. Our understanding of the interactions between the individual components draws substantially on various domains including agronomy, hydrology, economics, and others. We therefore envision assembling the components in a conceptual framework from multiple disciplines.
In summary, APNI proposes to lead the development and practical demonstration of the science that underpins the relationship between N, W and C and therefore enables a catalytic role for plant nutrition to sustain living agricultural landscapes. Key issues include:
• Nitrogen, water, and C are selected to represent changes in system entropy, or ecosystem sustainability (Addiscott, 1995), because NWC is expected to represent the system adequately. But the gap between the effects of these three factors and the total system is likely to be large.
• Thermodynamically, N, W and C make very unequal contributions towards system entropy. We treat them as equal, but we should perhaps scale them, as is done for CO2 equivalents.
• Sustainability must be represented at the system level. We deem this to be at least at the scale of landscapes since water movement within landscapes is the major source of variation in activity.
• Methods of estimating the conditions of N, W and C at the landscape scale need development, including a means of combining the three components into a single measure such as ternary landscape imagery (Fig. 1) used to represent changes in entropy.
• As a philosophical/moral issue that APNI science could help clarify, the consideration of entropy for agricultural systems may reveal that—while more organic and more ‘leak-proof’ systems are superior to those that require substantial external inputs—all agricultural systems increase entropy and are, theoretically speaking, unsustainable. The link to One Health, through analysis of a seamless system from input through to nutrition, may emerge in which APNI plays an important role.
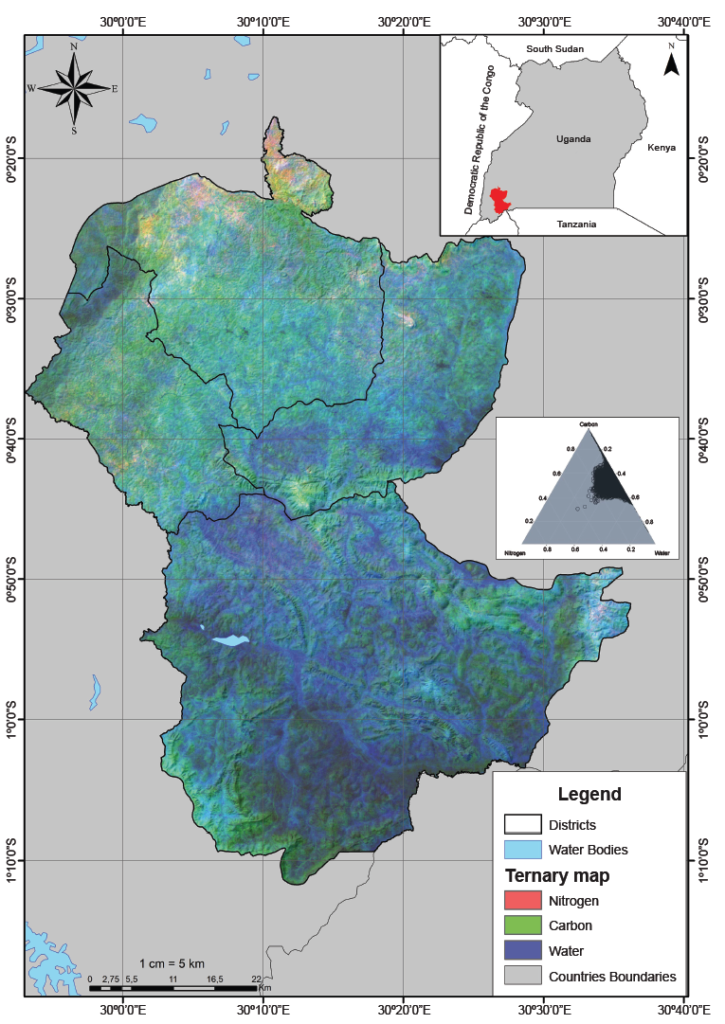
Nobel laureate Robert Solow (Solow, 1991) pointed out decades ago that growth based on the consumption of resources is, by definition, unsustainable, and largely a consequence of the undervaluation of the resource on which it depends. This applies to land or consumption of any input that does not account for externalities (e.g., N fertilizer). The true basis of sustainability lies in the development of know-how, an effectively inexhaustible process since there are always ways to do things smarter.
Opportunity
The activities of APNI to develop pathways to sustainable production that not only show how to use nutrient inputs better (through the 4Rs), but also to evaluate such use within a sustainability framework will be a major milestone for APNI and partners. The opportunity lies in understanding living agricultural landscapes in such a manner that water, C and N can be managed jointly to:
• Reduce N losses from soil while increasing N inputs to support biomass productivity gain through 4R management sensitized to hydronomic (i.e., water management) zones.
• Maximize the productivity from blue (i.e., surface or groundwater reservoirs) and green water use, while preserving essential environmental flows and protecting humid environments.
• Increase C capture and retention, by reducing soil erosion losses, increasing biomass inputs to soil, and increasing infiltration and throughflow.
• Enhance interaction between the three components to improve water productivity, N use efficiency, and C sequestration.
Some specific examples of the opportunities that 4R-based plant nutrition can unlock within a NWC concept include:
1. Resilient sustainable intensification (SI). Intensification is needed throughout Africa. Starting as it is from a low base, the potential for SI is huge. However, a significant rise in inorganic fertilizer use is crucial to initiate system improvement and drive systemic change in Africa. Further, many question this narrow focus as the best strategy for African systems. Increased fertilizer use may be necessary but insufficient to create the systemic change towards the resilient food systems that an estimated 33 million smallholder farmers need to implement. The role of NWC is to ensure that intensification of yield and water productivity is paralleled by a sustained or increased C budget, and without disproportionate increases in emissions of GHG from added N. NWC, therefore, broadens insight beyond yield within a coherent framework.
2. Smallholder-focused change. Smallholder farmers (SHFs) carry the risk of economic loss accompanied with higher fertilizer use, which is greater than might first appear in well-managed trial sites. Notwithstanding the overwhelming technical evidence supporting fertilizer use, such risks are greater in Africa than in areas with well-developed supply chains, markets, and financial institutions. SHFs manage multiple assets together, and judge how to increase productivity without jeopardizing other attributes of their systems, such as soil health, valued non-productive areas, and cover crops. The NWC model provides broader insight into biological sustainability than conventional methods and supports a view closer to the SHF perspective. NWC therefore supports farmer-centric decision making by assembling the key components of farming system change.
3. A measure that balances productivity growth with the need to protect soil health. Current discourses around soil health point out that this and several other concepts of sustainable agriculture (including conservation agriculture) are poorly defined, hence a risky basis for promoting development. However, scientists need to support change by reducing the structural uncertainty that obstructs it. The NWC concept therefore provides a rational, if still incomplete, basis on which to evaluate production landscapes.
Implementation
Agronomy that aims to implement the NCW Framework likely requires two innovative aspects that support targeted investment in crop nutrition at integrated scales (i.e., from continental to farm levels). Firstly, a top-down approach using data analyses to tackle the uncertainty connected to fertilizer use. Data sources include soil and socio-economic data, APNI legacy data, and high-resolution imagery. All data is interpreted through interdisciplinary science for 4R solutions, based on decades of agronomic insight from APNI. Secondly, a bottom-up approach captures on-farm innovation in 4R-driven improvements that create value across the chain. Viability is assessed through testing and revision.
To operationalize the NWC concept on the ground, strategic business intelligence is used for large-scale analysis to overcome uncertainties in crop nutrient response, return on investment, economic and social preparedness, and the impact of climate change. This cropping system analysis is a tool to identify specific constraints and improve components in production systems in target countries and regions. In addition, living labs promote site-specific solutions addressing SHF production expectations, resilience, intensification, GHG balance, C and N management, and water productivity. First approximations guide the right source formulations, foster farmer-centric innovation through networks for accelerated learning, and enable regions to adapt, fine-tune and adopt the right rate, time, and placement of nutrients. The NWC framework is proposed as a systemic approach that threads innovative agronomy and 4R from the regional scale to the cropping systems scale.
Dr. Mutegi is Senior Scientist, APNI, Nairobi, Kenya. e-mail: j.mutegi@apni.net. Ms. Mouamine is a Ph.D. Student, Agricultural Innovation and Technology Transfer Center (AITTC), College of Sustainable Agriculture and Environmental Sciences (CAES), Mohammed VI Polytechnic University (UM6P), Ben Guerir, Morocco. Dr. Boulal is Senior Scientist, APNI, Settat, Morocco. Dr. Amouzou is Scientist, APNI, Yamoussoukro, Cote d’Ivoire. Mr. Valencia is a M.Sc. Student at the Institute of Environmental Sciences, Kazan Federal University, Kazan, Russia, Dr. Oberthür is Director of Business and Partnerships, APNI, Ben Guerir, Morocco. Dr. Cook is Adjunct Professor, Murdoch University, Perth Australia.
Cite this article
Mutegi, J., Mouamine, I., Boulal, H., Amouzou, K.A., Valencia, B., Oberthür, T., Cook, S. 2023. The Role of 4R Plant Nutrition in Living Agricultural Landscapes: Developing the Nitrogen Water Carbon Nexus, Growing Africa 2(2): 2-7. https://doi.org/10.55693/ga22.HAJW5286
REFERENCES
Addiscott, T.M. 1995. Entropy and sustainability. Eur. J. Soil Sci., 46(2):161-168.
Cai, X., et al. 2013. Producing more food with less water in a changing world: assessment of water productivity in 10 major river basins. In, Water, food, and poverty in river basins, 280-300. Routledge.
Erkossa, T., Haileslassie, A,, Amede, T. 2015. Agricultural water productivity across landscape positions and management alternatives. Tropentag Conference on Management of Land Use Systems for Enhanced Food Security, Berlin, Germany, 4p.
Hengl, T., et al. 2021. African soil properties and nutrients mapped at 30 m spatial resolution using two-scale ensemble machine learning. Sci. Rep. 11: 6130.
Hengl, T., et al. 2017. SoilGrids250m: Global gridded soil information based on machine learning. PLoS ONE 12(2): e0169748.
ILRI. 2022. Amidst growing population pressures, who will feed Africa? https://www.ilri.org/news/amidst-growing-population-pressures-who-will-feed-africa.
Koutroulis, A.G., et al. 2019. Global water availability under high-end climate change: A vulnerability based assessment. Global and Planetary Change 175:52-63.
Kray, H., Shetty S., Colleye P.O. 2022. Three challenges and three opportunities for food security in Eastern and Southern Africa. World Bank Blogs. https://blogs.worldbank.org/africacan/three-challenges-and-three-opportunities-food-security-eastern-and-southern-africa.
Meena, R.S., Lal, R., Yadav, G.S. 2020. Long-term impact of topsoil depth and amendments on carbon and nitrogen budgets in the surface layer of an Alfisol in Central Ohio. Catena 194:104752.
Mutengwa, C.S., Mnkeni, P., Kondwakwenda, A. 2023. Climate-smart agriculture and food security in southern Africa: A review of the vulnerability of smallholder agriculture and food security to climate change. Sustainability 15(4):882.
Mutuku, M.M., et al. 2017. Factors that influence adoption of integrated soil fertility and water management practices by smallholder farmers in the semi-arid areas of eastern Kenya. Trop. Subtrop. Agroecosys. 20(1):141-153.
Ozor, N., Umunnakwe, P.C., Acheampong, E. 2013. Challenges of food security in Africa and the way forward. Development 56(3):404-411.
Schut, A.G., Giller, K.E. 2020. Sustainable intensification of agriculture in Africa. Front. Agric. Sci. Eng., 7(4):371-375.
Shrestha, E., et al. 2012. The carbon footprint of water management policy options. Energy Policy 42: 201-212.
Solow, R. 1991. Sustainability: An Economist’s perspective. The eighteenth J. Seward Johnson lecture. Woods Hole, MA: Woods Hole Oceanographic Institution.
The African Climate Foundation. 2023. African food systems transformation collective. https://africanclimatefoundation.org/news_and_analysis/african-food-systems-transformation-collective
Tilman, D., et al. 2011. Global food demand and the sustainable intensification of agriculture. Proc. Nat. Acad. Sci. 108(50): 20260-20264.
UN population. 2022. Department of economic and social affairs. Population division. World population prospects. https://population.un.org/wpp/Download/Standard/Population/
WRI. 2001. Building vital landscapes for sustainable and resilient growth in Africa. https://www.wri.org/wri-africa/building-vital-landscapes.
Zingore, S., Johnston, A. 2013. The 4R Nutrient Stewardship in the context of smallholder Agriculture in Africa. In, Vanlauwe, B., Blomme, G., Van Asten, P. (eds.), Agro-ecological Intensification of Farming Systems in the East and Central African Highlands, Earthscan, UK: 77-84.